Bioelectronics
Bioelectronics is a multidisciplinary field that combines biology and electronics engineering to create devices capable of monitoring or controlling biological processes. It involves integrating electronic components, such as sensors and circuits, with biological systems, such as cells and tissues.
Common bioelectronic devices include biomedical sensors, energy storage devices and harvesters, implantable devices, and soft robotics [1]. These technologies are utilized in monitoring glucose level for medical diagnostics, in therapeutic devices for drug delivery, and in neuroprosthetics for restoring lost functions, among others.
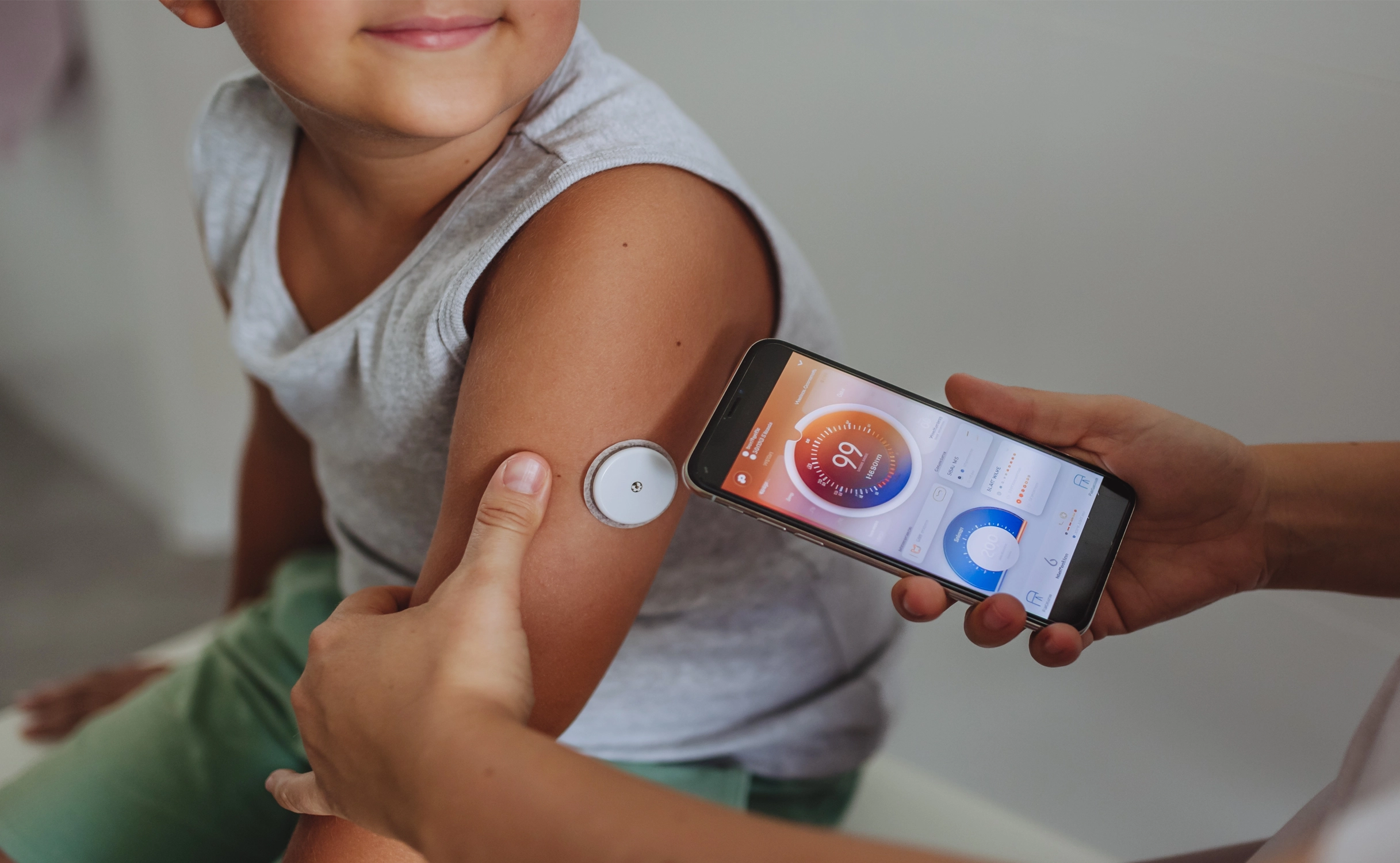
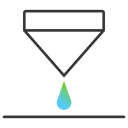
- Medical industry
- Prosthetics
- Pharmaceutical industry
- Consumer electronics
- Silver-based inks
- Gold-based inks
- Copper-based inks
- Carbon-based inks
- Polymer-based dielectric inks
- Ceramic-based dielectric inks
- Polydimethylsiloxane (PDMS)
- Polycarbonate (PC)
- Polyethylene terephthalate (PET)
- Hydrogels
- Silicon
- Fabric
Our Applications
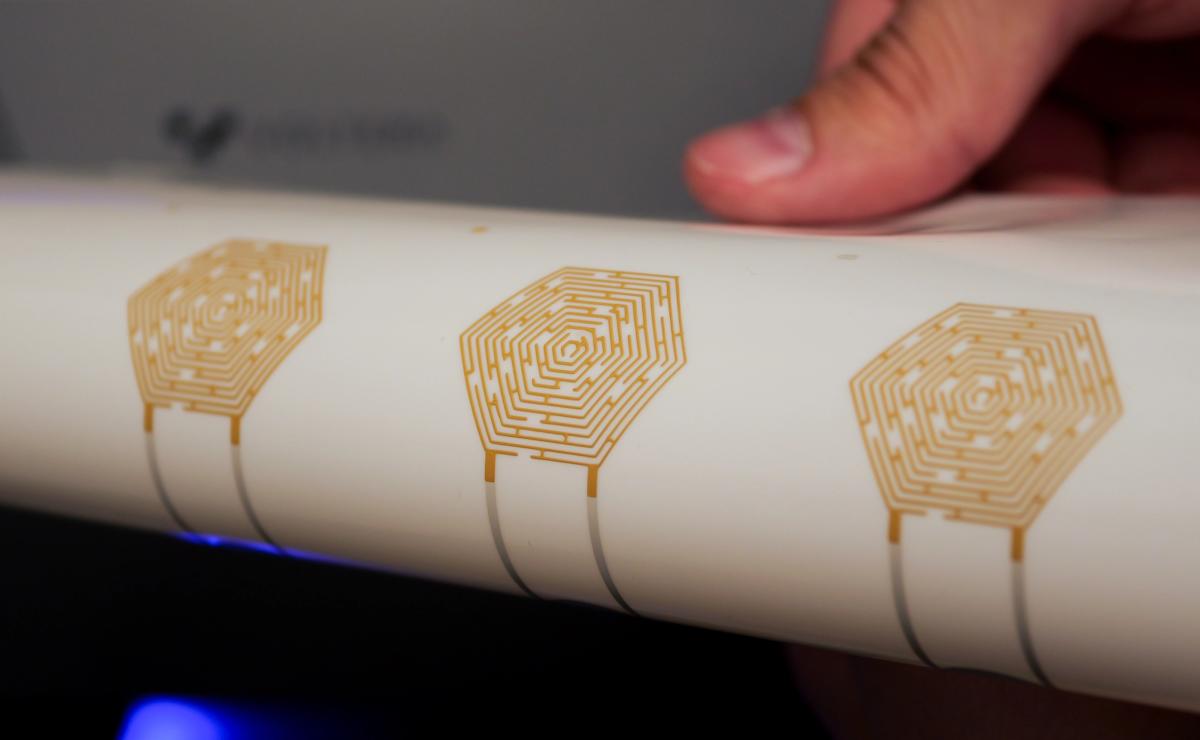
Printing ECG Electrodes with Biocompatible Gold Ink on TPU
This project demonstrates how we validated the effectiveness of printing ECG electrodes on TPU using biocompatible gold ink and stretchable silver ink.